Hormones, Homeostasis, and Behavior
- Published28 Oct 2022
- Author Diane A Kelly
- Source BrainFacts/SfN
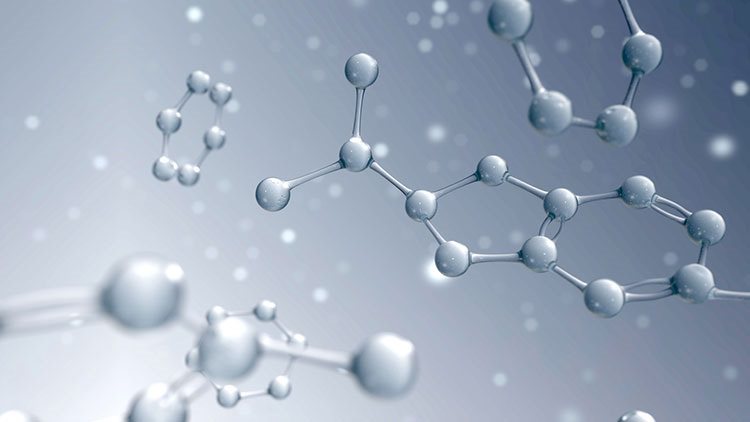
Parents often blame their teenager’s unpredictable behavior on hormones, but those molecules play a crucial role in the brain. Neurons can quickly deliver the brain’s messages to precise targets in the body. Hormones, on the other hand, deliver messages more slowly but can affect a larger set of tissues, producing large-scale changes in metabolism, growth, and behavior.
The brain is one of the tissues that “listens” for hormonal signals — neurons throughout the brain are studded with hormone receptors — and the brain’s responses play an important part in regulating hormone secretion and changing behaviors to keep body systems in equilibrium. The brain regions involved in hormone release are called the neuroendocrine system.
The hypothalamus oversees the production and release of many hormones through its close ties to the pituitary gland. The paraventricular and supraoptic nuclei of the hypothalamus send axons into the posterior part of the pituitary gland; activation of specific neurons releases either vasopressin or oxytocin into capillaries within the pituitary.
Both molecules act as neurotransmitters inside the brain, but they are also hormones that affect distant tissues of the body. Vasopressin (also called antidiuretic hormone) increases water retention in the kidneys and constricts blood vessels (vasoconstriction). Oxytocin promotes uterine contractions during labor and lactation during nursing.
Other hypothalamic regions send axons to a capillary-rich area above the pituitary called the median eminence. When these neurons are activated, they release their hormones into the blood. These releasing (and inhibiting) hormones travel through local blood vessels to the anterior pituitary, where they trigger (or inhibit) secretion of a second specific hormone.
Of the seven anterior pituitary hormones, five are trophic hormones — these travel in the bloodstream to stimulate activity in specific endocrine glands (thyroid, adrenal cortex, ovaries, etc.) throughout the body. The remaining two hormones act on non-endocrine tissues. Growth hormone stimulates the growth of bone and soft tissues, and prolactin stimulates milk production in the breasts. Hormones released from the anterior pituitary influence many aspects of life such as growth, emotion, cellular metabolism, hunger, thirst, stress, and the physiology of reproduction.
Many hormones produced by the pituitary and its target endocrine glands affect receptors inside the brain — thus, these hormones can alter neuronal function and gene transcription in the hypothalamus. The effect is to reduce the amount of hormones released by the hypothalamus when those circuits become active. These negative feedback loops enable precise doses of hormones to be delivered to body tissues and ensure that the hormone levels are narrowly regulated.
One of these three-hormone cascades regulates reproduction in mammals. Its underlying pattern is the same in both sexes: 1) gonadotropin-releasing hormone (GnRH) from the hypothalamus makes the anterior pituitary release 2) luteinizing hormone (LH) and follicle stimulating hormone (FSH), which in turn make the gonads secrete 3) sex hormones and start the development of mature eggs or sperm.
Sex hormones, in turn, attach to receptors in the hypothalamus and anterior pituitary, and modify the release of the hypothalamic and pituitary hormones. However, sex hormones regulate these feedback loops differently in males and females.
Male sex hormones induce simple negative feedback loops that reduce the secretion of GnRH, FSH, and LH. The interplay among these hormones creates a repetitive pulse of GnRH that peaks every 90 minutes. The waxing and waning of GnRH keeps testosterone levels relatively steady within body tissues, maintains male libido, and keeps the testes producing new sperm each day.
Female feedback patterns are more complex. Over the course of the month-long menstrual cycle, female sex hormones exert both positive and negative feedback on GnRH, FSH, and LH.
When circulating levels of the female sex hormones estrogen and progesterone are low, rising FSH levels trigger egg maturation and estrogen production. Rising estrogen levels induce LH levels to rise. As the levels of female sex hormones rise, they exert negative feedback on FSH secretion, limiting the number of eggs that mature in a month, but positive feedback on LH, eventually producing the LH surge that triggers ovulation. After ovulation, high serum levels of sex hormones again exert negative feedback on GnRH, FSH, and LH, which in turn reduces ovarian activity. Levels of female sex hormones therefore decrease, allowing the cycle to start over again.
Many other hormones are not regulated by the pituitary gland but released by specific tissues in response to physiological changes. The brain contains receptors for many of these hormones but, unlike pituitary hormones, it does not directly regulate their secretion. Instead, when these hormones bind to receptors on neurons, they modify the output of neural circuits, producing behavioral changes that have homeostatic effects. One example of this is a pair of hormones called leptin and ghrelin.
Leptin and ghrelin change eating behavior by regulating food intake and energy balance. Both hormones affect hunger, and both are released in response to changes in an animal’s internal energy stores. However, they have different effects on the circuits they regulate.
Ghrelin keeps the body fed. Released by the wall of the gastrointestinal tract when the stomach is empty, ghrelin activates hunger circuits in the hypothalamus that drive a search for food. Once the stomach is full, ghrelin production stops, reducing the desire to eat.
In contrast, leptin helps maintain body weight within a set range. Leptin is produced by fat cells and is released when fat stores are large. When it binds to neurons in the hypothalamus, leptin suppresses the activity of hunger circuits and reduces the desire to eat. As fat stores are used up, leptin levels decline, driving behavior that makes an animal eat more often and replenish its fat stores.
CONTENT PROVIDED BY
BrainFacts/SfN
References
Aguilera, G. (2011). HPA axis responsiveness to stress: Implications for healthy aging. Experimental Gerontology, 46(2-3), 90–95. https://doi.org/10.1016/j.exger.2010.08.023
Balkaya, M., Prinz, V., Custodis, F., Gertz, K., Kronenberg, G., Kroeber, J., Fink, K., Plehm, R., Gass, P., Laufs, U., & Endres, M. (2011). Stress worsens endothelial function and ischemic stroke via glucocorticoids. Stroke, 42(11), 3258–3264. https://pubmed.ncbi.nlm.nih.gov/21921276/
Carpenter, R., Reddi, B. 2012. Neurophysiology: A Conceptual Approach, Fifth Edition. Hodder Arnold: London.
Costello, R. B., Lentino, C. V., Boyd, C. C., O'Connell, M. L., Crawford, C. C., Sprengel, M. L., & Deuster, P. A. (2014). The effectiveness of melatonin for promoting healthy sleep: a rapid evidence assessment of the literature. Nutrition Journal, 13, 106. https://doi.org/10.1186/1475-2891-13-106
Debono, M., Ghobadi, C., Rostami-Hodjegan, A., Huatan, H., Campbell, M. J., Newell-Price, J., Darzy, K., Merke, D. P., Arlt, W., & Ross, R. J. (2009). Modified-Release Hydrocortisone to Provide Circadian Cortisol Profiles. The Journal of Clinical Endocrinology & Metabolism, 94(5), 1548–1554. https://doi.org/10.1210/jc.2008-2380
Dulac, C. (2010). Brain function and chromatin plasticity. Nature, 465(7299), 728–735. https://doi.org/10.1038/nature09231
Fain, G.L., & O’Dell, T. J. 2014. Molecular and Cellular Physiology of Neurons, Second Edition. Harvard University Press: Cambridge.
Gu, H. F., Tang, C. K., & Yang, Y. Z. (2012). Psychological stress, immune response, and atherosclerosis. Atherosclerosis, 223(1), 69–77. https://doi.org/10.1016/j.atherosclerosis.2012.01.021
Harris, A., & Seckl, J. (2011). Glucocorticoids, prenatal stress and the programming of disease. Hormones and Behavior, 59(3), 279–289. https://doi.org/10.1016/j.yhbeh.2010.06.007
Hasan, K. M., Rahman, M. S., Arif, K. M., & Sobhani, M. E. (2012). Psychological stress and aging: role of glucocorticoids (GCs). Age (Dordrecht, Netherlands), 34(6), 1421–1433. https://doi.org/10.1007/s11357-011-9319-0
Houben, T., Coomans, C. P., & Meijer, J. H. (2014). Regulation of Circadian and Acute Activity Levels by the Murine Suprachiasmatic Nuclei. PLOS ONE, 9(10), e110172. https://doi.org/10.1371/journal.pone.0110172
Klok, M. D., Jakobsdottir, S., Drent, M. L. (2007). The role of leptin and ghrelin in the regulation of food intake and body weight in humans: a review. Obesity Reviews, 8(1), 21–34. https://pubmed.ncbi.nlm.nih.gov/17212793/
Peschel, N., & Helfrich-Förster, C. (2011). Setting the clock – by nature: Circadian rhythm in the fruitfly Drosophila melanogaster. FEBS Letters, 585(10), 1435–1442. https://doi.org/10.1016/j.febslet.2011.02.028
Pfeffer, M., Korf, H. W., & Wicht, H. (2018). Synchronizing effects of melatonin on diurnal and circadian rhythms. General and Comparative Endocrinology, 258, 215–221. https://doi.org/10.1016/j.ygcen.2017.05.013
Purves, D. 2008. Neuroscience, Fourth Edition. Sinauer Associates, Inc.
Reynolds, R. M. (2013). Glucocorticoid excess and the developmental origins of disease: Two decades of testing the hypothesis – 2012 Curt Richter Award Winner. Psychoneuroendocrinology, 38(1), 1–11. https://doi.org/10.1016/j.psyneuen.2012.08.012
Richards, J., & Gumz, M. L. (2012). Advances in understanding the peripheral circadian clocks. The FASEB Journal, 26(9), 3602–3613. https://doi.org/10.1096/fj.12-203554
Skinner, M. K. (2014). Environmental stress and epigenetic transgenerational inheritance. BMC Medicine, 12, 153. https://doi.org/10.1186/s12916-014-0153-y
Sollars, P. J., & Pickard, G. E. (2015). The Neurobiology of Circadian Rhythms. Psychiatric Clinics of North America, 38(4), 645–665. https://doi.org/10.1016/j.psc.2015.07.003
Stojanovich L. (2010). Stress and autoimmunity. Autoimmunity Reviews, 9(5), A271–A276. https://doi.org/10.1016/j.autrev.2009.11.014
Swanson, L. W. 2012. Brain Architecture. 2nd edition. Oxford University Press: Oxford.
Tataroglu, O., & Emery, P. (2014). Studying circadian rhythms in Drosophila melanogaster. Methods, 68(1), 140–150. https://doi.org/10.1016/j.ymeth.2014.01.001
Tawakol, A., Ishai, A., Takx, R. A., Figueroa, A. L., Ali, A., Kaiser, Y., Truong, Q. A., Solomon, C. J., Calcagno, C., Mani, V., Tang, C. Y., Mulder, W. J., Murrough, J. W.,Hoffmann, U., Nahrendorf, M., Shin, L. M., Fayad, Z. A., & Pitman, R. K. (2017). Relation between resting amygdalar activity and cardiovascular events: a longitudinal and cohort study. The Lancet , 389(10071), 834–845. https://doi.org/10.1016/S0140-6736(16)31714-7
What to Read Next
Also In Body Systems
Trending
Popular articles on BrainFacts.org