Stable Yet Plastic: The Brain’s Balancing Act
- Published10 May 2023
- Author Calli McMurray
- Source BrainFacts/SfN
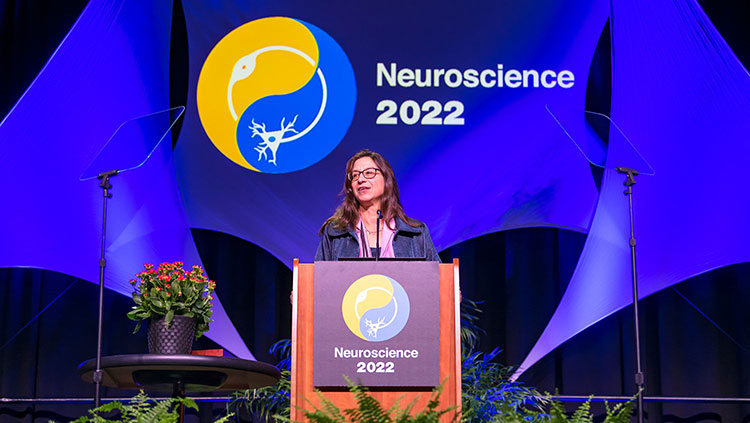
A fundamental concept of neuroscience was also, at one point, one of its biggest paradoxes. When two neurons send messages, or fire, at the same time, the connection between them strengthens. In turn, the neurons become more likely to fire again. And, increased firing, or excitabilty, makes the connection stronger still. Theoretically, this dynamic would charge forward until every connection in the brain reached a maximum strength, and no new information could be encoded: a conundrum called runaway excitation. But that’s not what happens. Gina Turrigiano has spent her career figuring out why.
Turrigiano, the Joseph Levitan Professor of Vision Science at Brandeis University and past Society for Neuroscience (SfN) president, is one of a few pioneers who opened the field of homeostatic plasticity, the study of how neurons tweak their own excitability to balance out circuit-wide changes in connection strength. Over the last three decades, Turrigiano has picked apart the mechanisms keeping neurons and circuits in check. Her work could one day reveal why brain function goes awry in diseases ranging from epilepsy to Alzheimer’s disease — and how to make it right again.
The Path to Homeostatic Plasticity
Turrigiano didn’t start her career intent on studying homeostasis. “I kind of serendipitously stumbled on a phenomenon that turned into an enormous research question that has driven my work ever since — this idea of self-regulation or homeostasis,” she says. As a graduate student and postdoctoral researcher, Turrigiano studied how the same neural circuit could act in different ways and initiate different behaviors in response to long-lasting neurochemicals called neuromodulators.
"And then weird stuff started to happen."
In one experiment as a postdoc at Brandeis University in 1994, she examined how the electrical properties of neurons in a feeding circuit changed in response to different neuromodulators. In the course of these experiments, she removed lobster neurons from their circuit and placed them in a cell culture dish to make them easier to study than when they are intact in a cluster of nerves. “And then weird stuff started to happen,” Turrigiano says.
In an intact circuit, the neurons fire together in a rhythmic pattern that depends on which neuromodulators are present. Isolated neurons don’t fire in the same pattern. But somehow, the single neurons in the dish began to fire in the same rhythmic patterns, without input from the rest of the circuit. “The individual neuron gained the ability to compensate for the things that it was missing,” she says. This looked a whole lot like a model of activity-dependent self-regulation that another postdoc in the lab was studying, leading her to try a key experiment demonstrating biological neurons can monitor their own activity levels and adjust their electrical properties to maintain a constant firing pattern.
After Turrigiano finished her postdoc, she started her own lab at Brandeis and pivoted to studying a new research question: how the synaptic connections between neurons avoid becoming too strong or too weak as neural circuits change during development and learning. In her newly formed lab, Turrigiano started to dig into whether neurons could homeostatically regulate synaptic properties, and if this self-regulation could explain the paradox of runaway excitation. Turrigiano turned to computational neuroscience for inspiration. Computational neuroscientists build artificial networks in which synaptic strengths can change in response to experience to store new information, but the artificial networks fall victim to runaway excitation. After a few rounds of sending messages, every connection in the network reached maximum strength and the system overloaded. The computational neuroscientists had to build in limits to the network, but it was unclear how biologically plausible these limits were.
Turrigiano wondered if a simple homeostatic rule allowing neurons to adjust synaptic strengths up or down to compensate for changes in firing could serve this stabilizing function. In a formative 1998 paper, Turrigiano and colleagues bathed cell cultures in a toxin that makes it harder for neurons to fire. If her theory was right, the neurons would ramp up the strength of each synapse to compensate for the decreased activity. That’s exactly what happened.
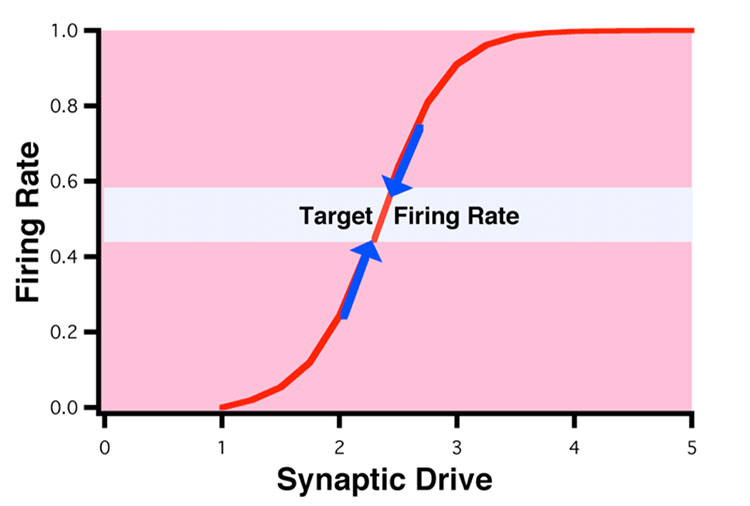
The neurons adjusted all of their excitatory synaptic strengths based on their firing rates, a phenomenon Turrigiano and her coauthors called synaptic scaling. The neurons behaved like a thermostat: They preferred a specific firing range and adjusted themselves when they strayed from range. “That became the first paper on synaptic scaling,” says Eve Marder, a biology professor at Brandeis University and Turrigiano’s postdoc advisor. “And that is what revolutionized the field.”
A Rich Vein
The paper made quite a splash: Computational and theoretical neuroscientists were excited, while synaptic plasticity researchers were doubtful. “There was definitely skepticism and pushback,” Turrigiano says. “And then, you know, I think what happened was people went in and they reproduced it.”
"There was definitely skepticism and pushback...what happened was people went in and they reproduced it.”
When different labs perform the same study and get the same results, scientists become more confident the results are accurate and not a fluke. Studies of homeostatic plasticity exploded across the field. “My lab realized what a rich vein this was,” Turrigiano says. Many labs joined Turrigiano’s in the quest to understand more specific mechanisms involved in synaptic scaling. “The whole synaptic strength field just jumped on this,” Marder says. Turrigiano and her collaborator Sacha Nelson compiled the nascent field’s major findings into an influential review in 2004, which drummed up even more excitement.
“Her seminal papers really built up and created the field,” says Richard Huganir, a neuroscience professor at Johns Hopkins University. “Just do a search on homeostatic plasticity, and you'll be impressed.”
Turrigiano then dug into a new question: Did behavioral state play a role in this type of plasticity? She didn’t think it would: They saw synaptic scaling in cell cultures, where there’s no such thing as being asleep or awake. But a 2016 and 2020 study in rats proved the hypothesis wrong. Downward regulation, or decreasing the firing rate, occurred only during sleep; upward regulation occurred only during wake.
Turrigiano is still exploring why the two directions of regulation are separated this way. “We can see the phenomenon, and I think that gives us a window into figuring out how to explain why,” she says, “but the why is not completely clear.”
Fixing Firing Gone Astray
As Turrigiano and other laboratories in the field of homeostatic plasticity learn more about how neurons regulate their firing, the potential applications draw nearer. If homeostatic mechanisms are impaired during development, it can unbalance brain activity over time. A circuit could eventually become hyperactive or hypoactive, which could contribute to autism spectrum disorder, or an out-of-whack firing range for a neuron could also lead to brain diseases like epilepsy and Alzheimer’s disease. “If we can understand the mechanisms, we could maybe figure out how to restore them in disease states,” Turrigiano says. “You could end up helping brain circuits get back in balance, even possibly in brains after they've already developed.”
Homing in on different cellular mechanisms could also help answer questions about how brain circuits remain stable as they change during learning, as well as how they remain flexible enough to do multiple jobs — the exact question Turrigiano studied as a graduate student.
“It’s sort of circling all the way back around to that question that motivated me at the very beginning of our research,” she says, “and we now understand enough that we can really begin trying to tackle it.”
CONTENT PROVIDED BY
BrainFacts/SfN
References
Hengen, K. B., Torrado Pacheco, A., McGregor, J. N., Van Hooser, S. D., & Turrigiano, G. G. (2016). Neuronal firing rate homeostasis is inhibited by sleep and promoted by wake. Cell, 165(1), 180–191. https://doi.org/10.1016/j.cell.2016.01.046
Torrado Pacheco, A., Bottorff, J., Gao, Y., & Turrigiano, G. G. (2021). Sleep Promotes Downward Firing Rate Homeostasis. Neuron, 109(3), 530–544. https://doi.org/10.1016/j.neuron.2020.11.001
Turrigiano, G., Abbott, L. F., & Marder, E. (1994). Activity-dependent changes in the intrinsic properties of cultured neurons. Science, 264(5161), 974–977. https://doi.org/10.1126/science.8178157
Turrigiano, G. G., Leslie, K. R., Desai, N. S., Rutherford, L. C., & Nelson, S. B. (1998). Activity-dependent scaling of quantal amplitude in neocortical neurons. Nature, 391(6670), 892–896. https://doi.org/10.1038/36103
Turrigiano, G. G., & Nelson, S. B. (2004). Homeostatic plasticity in the developing nervous system. Nature Reviews Neuroscience, 5(2), 97–107. https://doi.org/10.1038/nrn1327