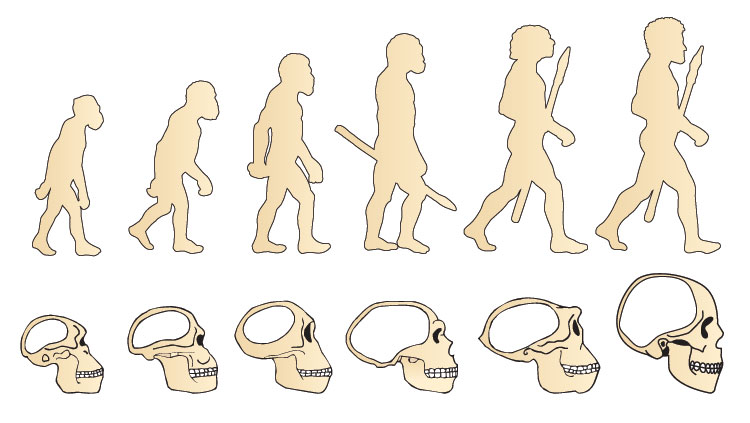
It’s hard to believe that our complex human brain evolved from a simple tube. The earliest vertebrates probably had brains much like the one in the modern lancelet Amphioxus — little more than a wide spot in the hollow nerve cord running down its back. But while the lancelet’s brain looks simple, it still contains specialized regions where neurons process specific kinds of information, like the presence of light or the chemicals drifting through the water. In its early development, the human brain began as a simple tube, and even today it is divided into the same kinds of regions as the brains of our ancestors.
In early vertebrates, the “brain” end of the nerve cord developed three distinct bulges as neurons were added, improving processing in sensory and motor reflex regions. These bulges became the forebrain, the midbrain, and the hindbrain. In the forebrain, the region able to detect chemicals expanded to form the olfactory bulbs, and with the evolution of image-producing eyes, light-sensing regions expanded and began processing more complex visual signals. The cerebellum appeared as the hindbrain and expanded the regions that control escape movements and orient the body in space. Both these functions are far more important to an actively swimming fish than to a sedentary lancelet buried in sand.
Regions that could rapidly process visual and auditory information and trigger appropriate escape, feeding, or mating behaviors also expanded in vertebrates. Over time, those new types of neurons made the forebrain balloon out, forming the right and left cerebral hemispheres. In early mammals, cortical tissues in the cerebrum and the cerebellum expanded even further, packing new neurons into layers and folds generating more complex tissues with increased processing power.
Adapted from the 8th edition of Brain Facts by Diane A. Kelly.
CONTENT PROVIDED BY
BrainFacts/SfN
References
Albuixech-Crespo, B., López-Blanch, L., Burguera, D., Maeso, I., Sánchez-Arrones, L., et al. (2017). Molecular regionalization of the developing amphioxus neural tube challenges major partitions of the vertebrate brain. PLOS Biology, 15(4): e2001573. https://doi.org/10.1371/journal.pbio.2001573
Barton, R. A., & Venditti, C. (2014). Rapid Evolution of the Cerebellum in Humans and Other Great Apes. Current Biology, 24(20), 2440–2444. https://doi.org/10.1016/j.cub.2014.08.056
Bekkers, J. M. (2011). Pyramidal neurons. Current Biology, 21(24), PR975. https://doi.org/10.1016/j.cub.2011.10.037
Belkhiria, C., Driss, T., Habas, C., Jaafar, H., Guillevin, R., & de Marco, G. (2017). Exploration and Identification of Cortico-Cerebellar-Brainstem Closed Loop During a Motivational-Motor Task: an fMRI Study. The Cerebellum, 16, 326–339. https://doi.org/10.1007/s12311-016-0801-1
Bromfield, E. B., Cavazos, J. E., Sirven, J. I. (2006). An Introduction to Epilepsy, https://www.ncbi.nlm.nih.gov/books/NBK2508/
Carpenter, R., & Reddi, B. (2012). Neurophysiology: A Conceptual Approach, 5th edition. Hodder Arnold: London.
Castro, A., Becerra, M., Manso, M. J., & Anadón, R. (2015). Neuronal organization of the brain in the adult amphioxus (Branchiostoma lanceolatum): A study with acetylated tubulin immunohistochemistry. The Journal of Comparative Neurology, 523(15), 2211–2232. https://doi.org/10.1002/cne.23785
Clarke, L. E., & Barres, B. A. (2013). Emerging roles of astrocytes in neural circuit development. Nature Reviews Neuroscience, 14, 311–321. https://doi.org/10.1038/nrn3484
Fain, G. L., & O’Dell T. J. (2014). Molecular and Cellular Physiology of Neurons, 2nd edition. Harvard University Press: Cambridge.
Forger, N. G. (2016). Epigenetic mechanisms in sexual differentiation of the brain and behaviour. Philosophical Transactions of the Royal Society B: Biological Sciences, 371(1688), 20150114. https://doi.org/10.1098/rstb.2015.0114
Frohlich, F. (2016). Network Neuroscience, 1st edition. Academic Press: London.
Guo, J. U., Ma, D. K., Mo, H., Ball, M. P., Jang, M. H., Bonaguidi, M. A., Balazer, J. A., Eaves, H. L., Xie, B., Ford, E., Zhang, K., Ming, G. L., Gao, Y., & Song, H. (2011). Neuronal activity modifies the DNA methylation landscape in the adult brain. Nature Neuroscience, 14, 1345–1351. https://doi.org/10.1038/nn.2900
Hammond, C. (2014). Cellular and Molecular Neurophysiology, 4th edition. Academic Press.
Human Brain. (2017). Allen Brain Atlas. Allen Institute for Brain Science. https://human.brain-map.org/
Lee, A., Fakler, B., Kaczmarek, L. K., & Isom, L. L. (2014). More Than a Pore: Ion Channel Signaling Complexes. The Journal of Neuroscience, 34(46), 15159–15169. https://doi.org/10.1523/JNEUROSCI.3275-14.2014
Noback, C. R. et al (eds.). (2005). The Human Nervous System: Structure and Function, 6th edition. Humana Press: Totowa NJ.
O'Muircheartaigh, J., Keller, S. S., Barker, G. J., & Richardson, M. P. (2015). White Matter Connectivity of the Thalamus Delineates the Functional Architecture of Competing Thalamocortical Systems. Cerebral Cortex, 25(11), 4477–4489. https://doi.org/10.1093/cercor/bhv063
Peer, M., Nitzan, M., Bick, A. S., Levin, N., & Arzy, S. (2017). Evidence for Functional Networks within the Human Brain's White Matter. The Journal of Neuroscience, 37(27), 6394–6407. https://doi.org/10.1523/JNEUROSCI.3872-16.2017
Pyka, M., & Cheng, S. (2014). Pattern Association and Consolidation Emerges from Connectivity Properties between Cortex and Hippocampus. PLOS ONE, 9(1), e85016. https://doi.org/10.1371/journal.pone.0085016
Saladin, K. (2015). Anatomy & Physiology: The Unity of Form and Function, 7th edition. McGraw Hill: New York.
Schneider, G. E. (2014). Brain Structure and its Origins: in Development and in Evolution of Behavior and the Mind. MIT Press: Cambridge.
Sheng, M., Kim, E. (2011). The postsynaptic organization of synapses. Cold Spring Harbor Perspectives in Biology, 3(12), a005678. https://pubmed.ncbi.nlm.nih.gov/22046028
Sporns, O. (2013). Structure and function of complex brain networks. Dialogues in Clinical Neuroscience, 15(3), 247–262. https://doi.org/10.31887/DCNS.2013.15.3/osporns
Verberne, A. J., Sabetghadam, A., & Korim, W. S. (2014). Neural pathways that control the glucose counterregulatory response. Frontiers in Neuroscience, 8(38). https://doi.org/10.3389/fnins.2014.00038
Wells, R. B. (2005). Cortical Neurons and Circuits: A Tutorial Introduction. https://webpages.uidaho.edu/rwells/techdocs/Cortical%20Neurons%20and%20Circuits.pdf