Meet the Inventors of Two-Photon Microscopy
- Published7 Dec 2021
- Author Andrew Meissen
- Source BrainFacts/SfN
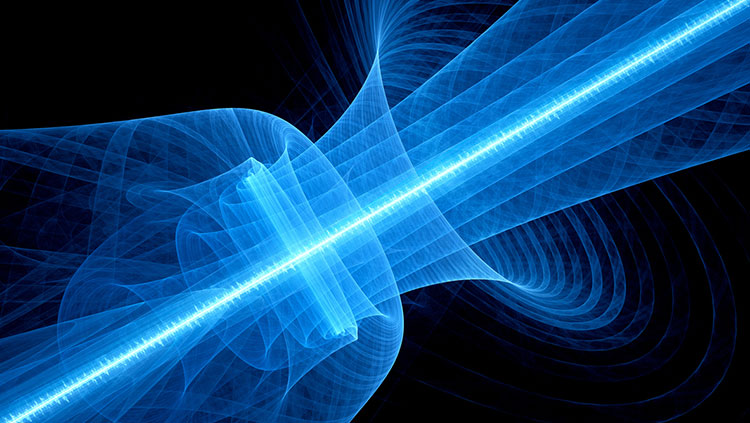
At the height of the Great Depression, Maria Goeppert Mayer submitted her doctoral thesis to Göttingen University in Germany. Her work suggested if a molecule absorbed two photons within very rapid succession, the photons could trigger an excited state in the molecule. Her idea required laser technology to test, so the scientific implications of this excited state were a mystery. So, Mayer moved on to other physics problems, ultimately collaborating with J. Hans D. Jensen to make Nobel Prize-winning discoveries on atomic structure (split with Eugene Paul Wigner) in 1963.
Around the same time Mayer was being honored with a Nobel Prize, her predicted two-photon excitation effect was confirmed experimentally in work that would later lead to the development of two-photon microscopy. The technique deploys the two-photon effect to generate high-quality images of cells. Fluorescent molecules called fluorophores emit light when they are excited via the two-photon effect. Injecting fluorophores into a slice of brain tissue allows researchers to send pulses of light into the tissue in order to gain sharper images of what they actually want to see.
Other fluorescent imaging techniques were available before two-photon microscopy. However, they didn’t produce highly detailed images of 3D structures because background fluorescence from outside the plane of focus would obscure the signal of interest.
In the 1980s, laser physicists at Cornell University were working next to scientists who were interested in microscopy. Winfried Denk was one of these scientists. Realizing the two-photon effect could be used to generate images with high resolution and contrast, he used high-powered lasers with just the right wavelength and structure of light pulses to create the first two-photon images.
Years later at Bell Labs, Denk, alongside David Tank, Karel Svoboda, and others realized two-photon microscopy is especially useful for fluorescence imaging in intact tissues, allowing scientists to see images of single neurons and even synapses in the intact brain. It worked well, but they encountered a problem: the method required researchers to gently inject fluorescent dye into the targeted brain tissue. It’s an imprecise method that can damage tissue you are most interested in looking at.
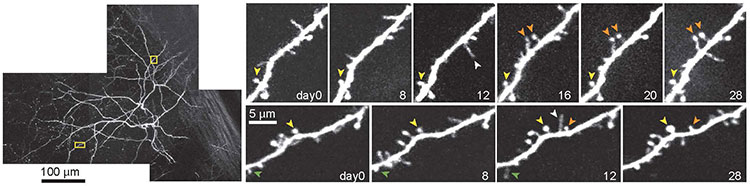
That problem was resolved with the discovery of the green fluorescent protein — a protein from jellyfish that glows green when exposed to certain wavelengths of light. Researchers now use genetic engineering to put green fluorescent protein or other fluorescent molecules into specific cell types, allowing them to see neurons — or whatever structure they genetically engineered to radiate green under the microscope — fully preserved. “That was a perfect marriage,” Svoboda says. “It really led to an explosion of the use of these methods in thousands of laboratories.”
In 2015, Denk, Svoboda, Arthur Konnerth and David W. Tank were awarded the prestigious Brain Prize by the Lundbeck Foundation for the development of two-photon microscopy. BrainFacts.org spoke with Denk and Svoboda about two-photon microscopy in the field and where their research is headed now.
How has your life and work changed since receiving the prize?
“The big advantage of these prizes is … one can get a little more freedom to do what one wants to do,” Denk says.
After receiving the Brain Prize, Denk wanted to extend his imaging work to investigate the origins of the nervous system’s many signals. Neurons receive signals from other cells, but where do those signals come from? “That’s really the question that ultimately will, I think, tell us how computation in the brain works.”
To do this, he needed a higher resolution than what could be provided by light microscopes. He turned to 3D electron microscopy so he could diagram the wiring of animals like mice and songbirds.
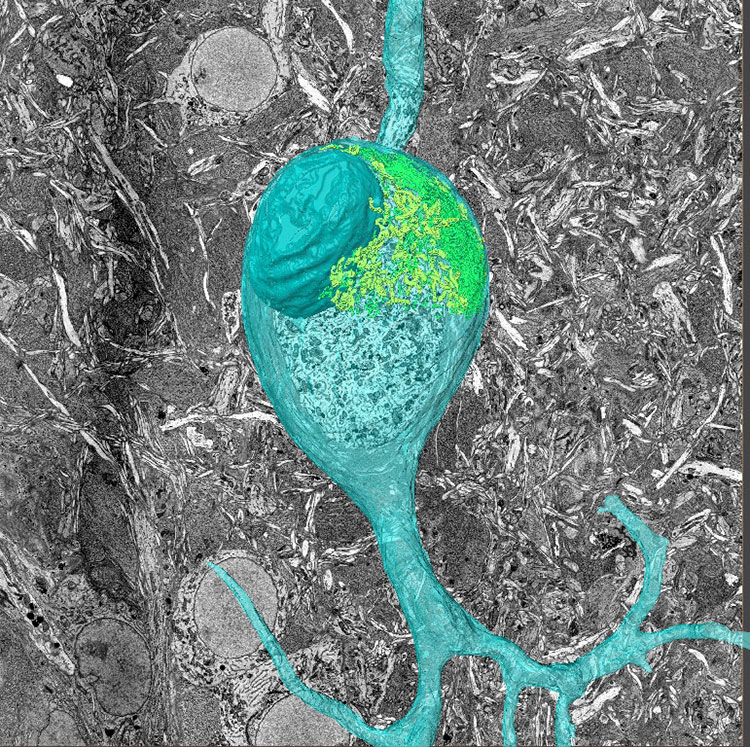
“We work on songbirds because they have well-codified behavior. Now in an intense collaboration with a former Bell Labs colleague of mine, Michale Fee at MIT, we are trying to figure out how exactly the birds learn” and where the information they learn is stored in their brains, Denk says. By viewing the neural structure underlying songbirds’ behaviors such as songs, scientists can gain a broader understanding of how the brain structures itself in general.
Svoboda is slated to be the director of the new Neural Dynamics division of the Allen Institute in Seattle, Wash. At the start of 2022, he will commence a 10- to 15-year effort to measure how neural circuits process information and produce behavior. The focus will be on measuring activity in neural circuits on a brain-wide scale and interpret how neurons work in the context of defined cell types.
Due to recent advancements in electrophysiology, Svoboda plans to use electrode technologies to extract details from individual neurons. Understanding neural activity in defined cell types will help scientists understand how neural circuits process information. “One approach that we’re trying to take is to try to learn how specific cell types differ in the kind of electrical images they produce on these new electrodes so that we can recognize specific neuron types using electrophysiology.”
Every neuron in the brain is different, but many neurons share large, noticeable differences. Some neurons have long spindly projections; others have short projections and readily snuggle up to other neurons. Researchers identify neuronal cell types genetically through RNA analysis and visually through microscopy.
What are the implications of your work for the field and for human health?
Two-photon microscopy and its subsequent discoveries have been used in many preclinical studies, from those seeking to combat neurodegeneration to other studies assessing the function of drugs, Svoboda says. There have been a number of non-neuroscience implications as well; certain studies of tumor development have been enabled by two-photon microscopy.
“I think going toward the cell-types level of understanding fundamental processes in the brain could be very promising in discovering methods to modulate neural function.” These new methods might help treat neuropsychiatric disorders, Svoboda adds.
Denk mentions, “I should say that I’ve retired my two-photon microscopes quite a while ago because I’ve made a major transition in my interests.” Inspired by his time imaging neural activity, he became interested in the foundational wiring for this activity. “What activity imaging showed me is how important it is to understand the structure underneath the activity.”
He had a problem with his two-photon microscopes: the resolution of light-based microscopes can’t go high enough to see fine fibers such as axons. In response, he developed a technique called Serial Block Face Scanning Electron Microscopy which creates a 3D image by sequentially slicing off thin layers of a tissue block, scanning the surface, and uploading the data. When the slices are recombined a 3D image emerges. When analyzing a whole mouse brain, the scanned pictures can be stitched back together to form an accurate diagram of neural wiring.
Like having a map, Denk explains that understanding structure can help us anticipate how to handle other scientific problems down the road. “In the end, we want to be able to know and understand better what certain perturbations” like surgery, chemicals, or light do to neural structures. Knowing how the nervous system is shaped can bestow scientists a type of grounding to keep track of their progress.
Denk is hoping to help make a neural circuit diagram for the songbird and other animals such as the mouse in the next decade. “If we can figure out these wiring diagrams, I think this will make a huge impact, probably more so than the two-photon microscope ever had.”
CONTENT PROVIDED BY
BrainFacts/SfN
References
Maria Goeppert Mayer – Biographical. (2021). NobelPrize.org. https://www.nobelprize.org/prizes/physics/1963/mayer/biographical/
Kubota, Y., Sohn, J., Hatada, S., Schurr, M., Straehle, J., Gour, A., ... & Kawaguchi, Y. (2018). A carbon nanotube tape for serial-section electron microscopy of brain ultrastructure. Nature communications, 9(1), 1-15. https://doi.org/10.1038/s41467-017-02768-7
Tasic, B., Yao, Z., Graybuck, L. T., Smith, K. A., Nguyen, T. N., Bertagnolli, D., ... & Zeng, H. (2018). Shared and distinct transcriptomic cell types across neocortical areas. Nature, 563(7729), 72-78. https://doi.org/10.1038/s41586-018-0654-5