Firing Messages: Ion Channels and Action Potentials
- Reviewed14 Nov 2022
- Author Diane A. Kelly
- Source BrainFacts/SfN
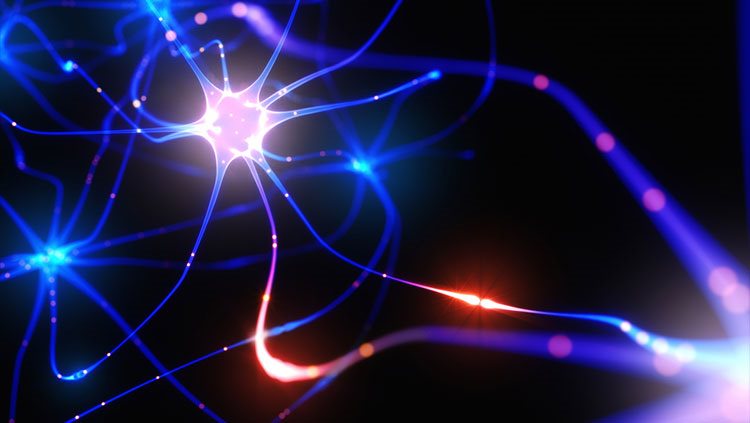
Ions are electrically charged atoms that can only cross a neuron’s cell membrane through tunnel-like proteins called ion channels. These tunnel-like proteins act like gates, allowing some ions to enter or leave the cell, but keeping others out. Ions that enter or leave the cell change the voltage difference across the membrane. This change in voltage influences the neuron’s likelihood of generating an electrical signal.
In mammals, the voltage difference across the membrane of a resting neuron is around -70 millivolts (mV), more negative inside the cell than on its outer surface. That membrane potential is affected by signals arriving from other neurons in its circuit, which can make the membrane potential less negative (depolarized) or more negative (hyperpolarized) by opening ion channels in the dendrites. If the sum of all the signals at the dendrites rises to match the membrane’s threshold voltage, a series of voltage-sensitive ion channels opens automatically, triggering an electrical impulse called an action potential, which moves down the axon towards the next neuron in the circuit.
Adapted from the 8th edition of Brain Facts by Diane A. Kelly.
CONTENT PROVIDED BY
BrainFacts/SfN
References
Barton, R. A., & Venditti, C. (2014). Rapid Evolution of the Cerebellum in Humans and Other Great Apes. Current Biology, 24(20), 2440–2444. https://doi.org/10.1016/j.cub.2014.08.056
Bekkers, J. M. (2011). Pyramidal neurons. Current Biology, 21(24), PR975. https://doi.org/10.1016/j.cub.2011.10.037
Belkhiria, C., Driss, T., Habas, C., Jaafar, H., Guillevin, R., & de Marco, G. (2017). Exploration and Identification of Cortico-Cerebellar-Brainstem Closed Loop During a Motivational-Motor Task: an fMRI Study. The Cerebellum, 16, 326–339. https://doi.org/10.1007/s12311-016-0801-1
Bromfield, E. B., Cavazos, J. E., Sirven, J. I. (2006). An Introduction to Epilepsy, https://www.ncbi.nlm.nih.gov/books/NBK2508/
Carpenter, R., & Reddi, B. (2012). Neurophysiology: A Conceptual Approach, 5th edition. Hodder Arnold: London.
Castro, A., Becerra, M., Manso, M. J., & Anadón, R. (2015). Neuronal organization of the brain in the adult amphioxus (Branchiostoma lanceolatum): A study with acetylated tubulin immunohistochemistry. The Journal of Comparative Neurology, 523(15), 2211–2232. https://doi.org/10.1002/cne.23785
Clarke, L. E., & Barres, B. A. (2013). Emerging roles of astrocytes in neural circuit development. Nature Reviews Neuroscience, 14, 311–321. https://doi.org/10.1038/nrn3484
Fain, G. L., & O’Dell T. J. (2014). Molecular and Cellular Physiology of Neurons, 2nd edition. Harvard University Press: Cambridge.
Forger, N. G. (2016). Epigenetic mechanisms in sexual differentiation of the brain and behaviour. Philosophical Transactions of the Royal Society B: Biological Sciences, 371(1688), 20150114. https://doi.org/10.1098/rstb.2015.0114
Frohlich, F. (2016). Network Neuroscience, 1st edition. Academic Press: London.
Guo, J. U., Ma, D. K., Mo, H., Ball, M. P., Jang, M. H., Bonaguidi, M. A., Balazer, J. A., Eaves, H. L., Xie, B., Ford, E., Zhang, K., Ming, G. L., Gao, Y., & Song, H. (2011). Neuronal activity modifies the DNA methylation landscape in the adult brain. Nature Neuroscience, 14, 1345–1351. https://doi.org/10.1038/nn.2900
Hammond, C. (2014). Cellular and Molecular Neurophysiology, 4th edition. Academic Press.
Human Brain. (2017). Allen Brain Atlas. Allen Institute for Brain Science. https://human.brain-map.org/
Lee, A., Fakler, B., Kaczmarek, L. K., & Isom, L. L. (2014). More Than a Pore: Ion Channel Signaling Complexes. The Journal of Neuroscience, 34(46), 15159–15169. https://doi.org/10.1523/JNEUROSCI.3275-14.2014
Noback, C. R. et al (eds.). (2005). The Human Nervous System: Structure and Function, 6th edition. Humana Press: Totowa NJ.
O'Muircheartaigh, J., Keller, S. S., Barker, G. J., & Richardson, M. P. (2015). White Matter Connectivity of the Thalamus Delineates the Functional Architecture of Competing Thalamocortical Systems. Cerebral Cortex, 25(11), 4477–4489. https://doi.org/10.1093/cercor/bhv063
Peer, M., Nitzan, M., Bick, A. S., Levin, N., & Arzy, S. (2017). Evidence for Functional Networks within the Human Brain's White Matter. The Journal of Neuroscience, 37(27), 6394–6407. https://doi.org/10.1523/JNEUROSCI.3872-16.2017
Pyka, M., & Cheng, S. (2014). Pattern Association and Consolidation Emerges from Connectivity Properties between Cortex and Hippocampus. PLOS ONE, 9(1), e85016. https://doi.org/10.1371/journal.pone.0085016
Saladin, K. (2015). Anatomy & Physiology: The Unity of Form and Function, 7th edition. McGraw Hill: New York.
Schneider, G. E. (2014). Brain Structure and its Origins: in Development and in Evolution of Behavior and the Mind. MIT Press: Cambridge.
Sheng, M., Kim, E. (2011). The postsynaptic organization of synapses. Cold Spring Harbor Perspectives in Biology, 3(12), a005678. https://pubmed.ncbi.nlm.nih.gov/22046028
Sporns, O. (2013). Structure and function of complex brain networks. Dialogues in Clinical Neuroscience, 15(3), 247–262. https://doi.org/10.31887/DCNS.2013.15.3/osporns
Verberne, A. J., Sabetghadam, A., & Korim, W. S. (2014). Neural pathways that control the glucose counterregulatory response. Frontiers in Neuroscience, 8(38). https://doi.org/10.3389/fnins.2014.00038
Wells, R. B. (2005). Cortical Neurons and Circuits: A Tutorial Introduction. https://webpages.uidaho.edu/rwells/techdocs/Cortical%20Neurons%20and%20Circuits.pdf